Maximising Power: Exploring Energy Density and Efficiency in Modern Batteries
- Vuong Loi Quang
- Mar 12, 2024
- 13 min read
Updated: Jul 13, 2024
In today's technology-driven world, the demand for high-performance batteries is soaring. This blog post explores energy density, battery efficiency improvements, and the energy-to-weight ratio. Discover how these factors maximise power and performance in modern batteries. Let's unlock the potential for a sustainable and efficient future.
How can high-energy-density batteries improve the performance of electronic devices?

High-energy-density batteries play a crucial role in enhancing the performance of electronic devices. By storing more energy compactly, these batteries provide a higher power-to-weight ratio, allowing electronic devices to operate for longer periods without frequent recharging.
The increased energy density enables electronic devices to have longer battery life, making them more convenient and reliable. With high-energy-density batteries, users can enjoy extended usage without interruptions, ensuring a seamless and uninterrupted experience.
Moreover, these batteries enable electronic devices to handle demanding tasks more efficiently. The higher energy density allows for increased power output, enhancing the performance of devices such as smartphones, laptops, and tablets. This means faster processing speeds, smoother multitasking, and improved overall performance.
In addition, high-energy-density batteries are essential for portable devices that require compact and lightweight power sources. The compact design allows for sleeker and more streamlined device designs, making them easier to carry and handle. Whether it's a slim smartphone or a lightweight wearable device, high-energy-density batteries contribute to the overall user experience by enabling smaller and more portable designs.
Furthermore, the improved performance of electronic devices powered by high-energy-density batteries also positively impacts sustainability. With longer battery life, the need for frequent battery replacements is reduced, leading to less waste and a smaller environmental footprint. This enhances electronic devices' overall lifespan and usability, making them more eco-friendly.
In summary, high-energy-density batteries bring significant advantages to electronic devices by enhancing their performance, extending battery life, enabling more efficient power output, supporting portable designs, and promoting sustainability. With these batteries, electronic devices become more powerful, reliable, and environmentally friendly, improving the overall user experience.
What are the key factors that determine battery efficiency improvements?
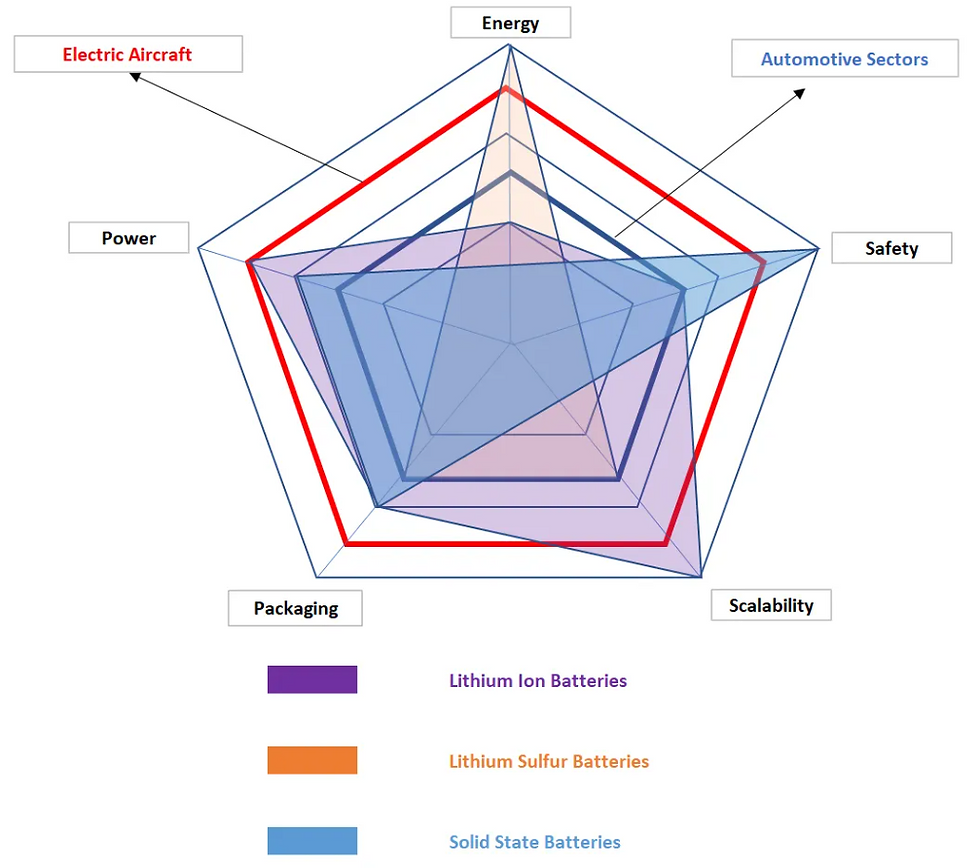
Several key factors determine battery efficiency improvements:
Chemical Composition: The choice of materials used in a battery's chemical composition greatly impacts its efficiency. Batteries with high energy density materials, such as lithium-ion batteries, have proven more efficient than traditional lead-acid batteries.
Design and Engineering: The design and engineering of a battery play a crucial role in its efficiency. Factors such as the arrangement and size of the battery cells, electrode design, and overall system architecture can significantly impact how efficiently the battery operates.
Temperature Management: Temperature has a significant impact on battery efficiency. Batteries perform best at optimum operating temperatures, and excessive heat or cold can decrease efficiency. Effective temperature management systems can help optimise battery performance and prolong its lifespan.
Charge and Discharge Rate: Battery efficiency is also influenced by the rate at which it is charged and discharged. Batteries that can be charged and discharged at higher rates without compromising performance are more efficient.
Battery Management Systems: Implementing advanced battery management systems can improve battery efficiency by monitoring and optimising various parameters such as voltage, current, and temperature. These systems help maximise the usable capacity of the battery and prevent overcharging or over-discharging, which can reduce efficiency.
Cycle Life: The number of charge-discharge cycles a battery can withstand without significant degradation also affects its efficiency. Batteries with longer cycle lives can retain their efficiency over extended periods, making them more desirable for various applications.
Maintenance and Care: Proper maintenance and care of batteries are essential for optimising their efficiency. This includes regular inspections, cleaning, and ensuring proper charging and storage practices as the manufacturer recommends.
By considering these key factors, battery manufacturers and users can work together to improve battery efficiency and achieve better overall performance.
How can advancements in the energy-to-weight ratio impact the development of electric vehicles?
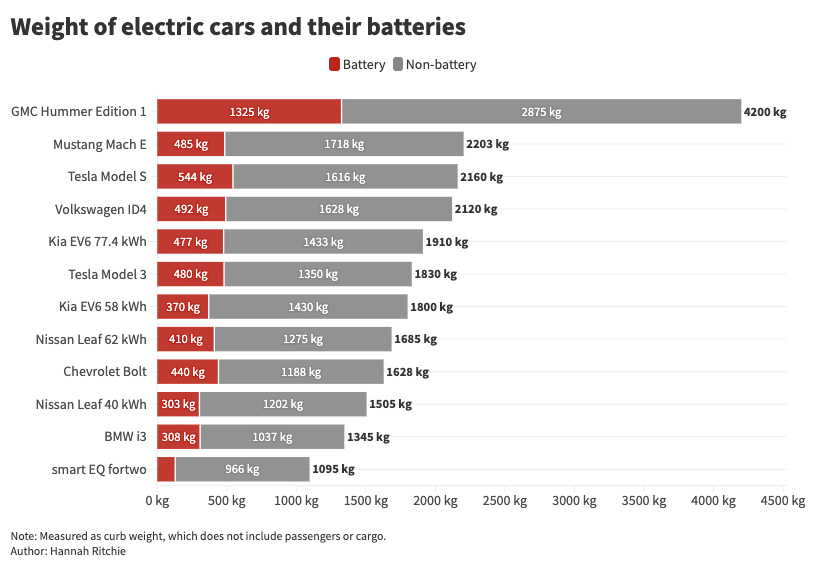
Advancements in the energy-to-weight ratio can have a significant impact on the development of electric vehicles (EVs). The energy-to-weight ratio measures how efficiently energy is stored in a vehicle's battery relative to its weight.
A higher energy-to-weight ratio means that a vehicle can store more energy in its battery for the same weight or have a lighter battery for the same amount of energy. This has several implications for electric vehicles:
Longer driving range: A higher energy-to-weight ratio enables electric vehicles to have a longer driving range. With more energy stored in the battery, the vehicle can travel greater distances before needing to recharge. This is especially important for electric vehicles, as one of the main concerns for consumers is range anxiety.
Smaller and lighter batteries: A higher energy-to-weight ratio allows for the development of smaller and lighter batteries. This reduces the vehicle's overall weight and frees up space, providing opportunities for improved designs and greater flexibility in vehicle architecture. Smaller and lighter batteries also increase efficiency, as the vehicle requires less energy to move its weight.
Faster charging times: Advancements in the energy-to-weight ratio can lead to faster charging times for electric vehicles. High-energy-density batteries can store more energy, so the vehicle can be charged more quickly. This improves convenience for electric vehicle owners and helps address the issue of limited charging infrastructure.
Sustainability: A higher energy-to-weight ratio can contribute to the overall sustainability of electric vehicles. By increasing energy storage capacity without adding significant weight, manufacturers can reduce the environmental impact of battery production and disposal. This supports the long-term viability and adoption of electric vehicles as a cleaner, more sustainable transportation solution.
In conclusion, advancements in energy-to-weight ratio play a crucial role in shaping the development of electric vehicles. They enable longer driving ranges, smaller and lighter batteries, faster charging times, and contribute to the overall sustainability of electric transportation. As researchers and engineers progress in this area, we can expect to see even more efficient and capable electric vehicles.
What are the current challenges in achieving higher energy density in batteries?
There are several key challenges that researchers and engineers are currently facing in their quest to achieve higher energy density in batteries.
Materials Limitations: One of the main challenges lies in finding suitable materials that can store and release more energy. Lithium-ion batteries dominate the market due to their high energy density, but they still have room for improvement. Researchers are exploring alternative materials, such as lithium-sulfur or lithium-air, which have the potential to offer higher energy densities.
Safety Concerns: As energy densities increase, safety concerns are associated with storing and releasing larger amounts of energy. Higher energy densities can lead to increased heat generation and potential thermal runaway, which poses risks of battery failure or even explosions. Developing battery technologies with enhanced safety features is a critical challenge that needs to be addressed.
Cycle Life and Degradation: Another significant challenge is increasing the cycle life of batteries, which refers to how many times they can be charged and discharged before their capacity significantly decreases. During charge and discharge cycles, the continuous chemical reactions within a battery can result in electrode degradation and capacity loss over time. Batteries' durability and longevity are crucial for achieving higher energy densities.
Cost and Scalability: The cost of battery production and the ability to scale up manufacturing are also important challenges. Higher energy density often requires more expensive materials and intricate manufacturing processes. Finding ways to reduce costs without compromising performance is essential to make high-energy-density batteries commercially viable on a larger scale.
Environmental Impact: Lastly, it is crucial to consider the environmental impact of batteries. The extraction and processing of raw materials needed for high-energy-density batteries can lead to environmental degradation and contribute to carbon emissions. Developing sustainable and recyclable battery technologies is crucial to minimise the ecological footprint associated with battery production and disposal.
In summary, achieving higher energy density in batteries involves addressing challenges related to materials, safety, cycle life, cost, scalability, and environmental impact. These challenges require ongoing research and innovation to pave the way for the development of advanced battery technologies that can meet the growing energy demands of the future.
How do improvements in battery efficiency contribute to sustainable energy storage solutions?
Improvements in battery efficiency play a crucial role in achieving sustainable energy storage solutions.
Battery efficiency refers to the ability of a battery to convert stored energy into electrical power with minimal losses. When batteries are more efficient, they can store and release energy more effectively, leading to several benefits for sustainable energy storage:
Enhanced Energy Storage Capacity: Higher battery efficiency means more energy can be stored within the same physical size of the battery. This enables greater energy storage capacity, allowing for the accumulation of electricity generated from renewable sources like solar or wind power.
Optimized Resource Utilization: Efficient batteries reduce energy waste during storage and retrieval. By minimising losses, a larger percentage of the stored energy can be utilised and converted into usable electricity. This maximises the utilisation of precious resources, reducing the environmental impact of energy production.
Extended Battery Lifespan: Improved battery efficiency helps mitigate factors that can degrade battery performance, such as heat generation and chemical degradation. When batteries operate more efficiently, they experience less stress, extending their lifespan. This reduces the need for frequent battery replacements, reducing waste and minimising environmental impact.
Economy and Affordability: Higher battery efficiency fosters cost-effective energy storage solutions. Efficient batteries require fewer raw materials to generate the same amount of usable energy, reducing production costs. As the economies of scale kick in, the cost of manufacturing and implementing energy storage systems decreases, making sustainable energy storage solutions more affordable and accessible in the long run.
Grid Stability and Resilience: Battery efficiency also contributes to grid stability and resilience. Efficient batteries offer fast response times and reliable energy delivery, making them suitable for balancing the variable energy generated by renewable sources. By storing excess energy during periods of high generation and releasing it during peak demand, these batteries help maintain a stable electricity supply.
In summary, improvements in battery efficiency are pivotal for sustainable energy storage solutions. They enable increased energy storage capacity, optimise resource utilisation, extend battery lifespan, enhance affordability, and contribute to grid stability. Investing in battery research and development is a key driver in transitioning towards a more sustainable and renewable energy future.
What role do materials science and engineering play in enhancing the energy-to-weight ratio in batteries?
Materials science and engineering play a crucial role in enhancing the energy-to-weight ratio in batteries. The energy-to-weight ratio refers to the amount of energy that can be stored in a battery relative to its weight. This ratio is key to determining the performance and efficiency of batteries, especially in applications where weight is a limiting factor, such as in electric vehicles and portable electronic devices.
To improve the energy-to-weight ratio, materials scientists and engineers focus on developing and optimising the materials used in battery construction. They work on identifying and designing materials that can store and release a lot of energy while maintaining a low weight. This involves a deep understanding of materials' physical and chemical properties and their interaction within the battery system.
One approach for enhancing the energy-to-weight ratio is developing advanced electrode materials. These materials are responsible for storing and releasing charge during the battery's operation. Materials scientists aim to discover new electrode materials or improve existing ones to increase their energy storage capacity while reducing weight. This could involve exploring new compounds, nanostructured materials, or composites combining multiple materials with complementary properties.
Another important aspect is the optimisation of the electrolyte, which is the medium that allows the flow of ions between the electrodes in a battery. Researchers look for electrolyte materials with high ionic conductivity, low resistance, and good stability. By improving the performance of the electrolyte, the energy transfer within the battery can be enhanced, leading to a higher overall energy-to-weight ratio.
Furthermore, materials scientists and engineers also focus on designing and engineering battery components to maximise the energy-to-weight ratio. This may involve optimising the architecture and structure of the battery, including using lightweight and durable materials for the battery case and current collectors. By reducing the weight of non-active components, the overall energy-to-weight ratio can be improved.
In summary, materials science and engineering significantly enhance the energy-to-weight ratio in batteries. Through developing advanced electrode materials, optimising the electrolyte, and using lightweight and efficient battery components, researchers strive to improve the energy storage capacity while reducing the weight of batteries, resulting in more efficient and lightweight energy storage solutions for various applications.
How can lithium-ion batteries be optimised for higher energy density and efficiency?
Lithium-ion batteries can be optimised for higher energy density and efficiency through several key strategies:
Material Selection: Choosing the right materials for the battery components is crucial. Researchers and manufacturers constantly explore new electrode materials, electrolytes, and separators to enhance energy storage capacity, efficiency, and stability.
Electrode Design: Optimizing the design of the electrodes can significantly improve battery performance. Increasing the electrode surface area, maximising active material loading, and optimising the electrode structure can lead to higher energy density and improved charge/discharge rates.
Cell Configuration: The arrangement and configuration of battery cells can impact energy density and efficiency. Engineering the cell architecture, such as stacking multiple cells in series or parallel, can increase the battery's overall capacity and power output.
Electrolyte Optimization: The electrolyte plays a crucial role in battery performance. Researchers are exploring various techniques to improve electrolyte conductivity, enhance stability, and enable higher operating voltages, thus increasing energy density and efficiency.
Battery Management Systems (BMS): Implementing advanced BMS can optimise battery performance by monitoring and controlling the charging and discharging processes. BMS ensures proper balance between cells, protects against overcharging or discharging, and prolongs the battery's lifespan.
Thermal Management: Efficient thermal management is essential for maintaining battery performance and preventing overheating. By implementing cooling systems or optimising the battery's thermal design, energy density and efficiency can be maximised while ensuring the safety and longevity of the battery.
Manufacturing Processes: Innovation in battery manufacturing can also contribute to higher energy density and efficiency. Technologies like roll-to-roll production, electrode coating techniques, and advanced quality control measures help to increase production yields and reduce costs.
By implementing these optimisation strategies, lithium-ion batteries can achieve higher energy density and efficiency, enabling longer-lasting and more powerful energy storage solutions for various applications, such as electric vehicles, renewable energy storage, portable electronics, and more.
What are some emerging technologies that could revolutionise the energy storage industry in terms of energy-to-weight ratio?
One emerging technology with great potential is the development of solid-state batteries. Unlike traditional lithium-ion batteries that use liquid electrolytes, solid-state batteries use a solid electrolyte material. These batteries could offer higher energy density, longer lifespan, and improved safety compared to conventional batteries.
Hydrogen fuel cells are another technology that could revolutionise energy storage. Fuel cells use hydrogen gas as a fuel source and convert it into electricity through a chemical reaction. Hydrogen fuel cells boast a high energy-to-weight ratio and can provide a continuous power source as long as hydrogen is supplied. They emit only water vapour as a byproduct, making them an environmentally friendly option.
Graphene supercapacitors are also an emerging technology with significant potential. Graphene, a single layer of carbon atoms arranged in a hexagonal lattice, has remarkable electrical and mechanical properties. Graphene supercapacitors can store energy efficiently and offer a higher power density than conventional batteries, making them ideal for applications requiring rapid energy discharge and recharge cycles.
Flywheel energy storage systems are gaining attention for high-capacity energy storage. These systems use a rotating flywheel to store kinetic energy, which can be converted back into electrical energy when needed. Flywheels have a high energy-to-weight ratio and deliver power instantly. They are long-lasting and require minimal maintenance.
Advancements in nanotechnology also hold the potential to revolutionise energy storage. Nanostructured materials for batteries and capacitors can enhance energy storage capabilities by increasing surface area, improving charge-discharge rates, and boosting overall performance. Nanotechnology offers the possibility of integrating energy storage devices directly into products like clothing and electronic devices, enabling seamless and efficient energy storage solutions.
In conclusion, solid-state batteries, hydrogen fuel cells, graphene supercapacitors, flywheel energy storage systems, and nanotechnology are all emerging technologies with the potential to revolutionise the energy storage industry in terms of energy-to-weight ratio. These advancements promise to deliver more efficient, durable, and environmentally friendly energy storage solutions, meeting the growing demand for clean and sustainable energy sources.
How do factors such as temperature, charge-discharge cycles, and electrode design affect the performance of high-energy-density batteries?
Factors such as temperature, charge-discharge cycles, and electrode design play a crucial role in determining the performance of high-energy-density batteries.
Temperature: The temperature at which a battery operates significantly impacts its performance. High temperatures can accelerate the rate of chemical reactions inside the battery, leading to faster degradation of the electrodes and a decrease in overall battery life. On the other hand, low temperatures can result in reduced ionic conduction, which can decrease the battery's ability to deliver power. Maintaining an optimal operating temperature is essential to ensure the longevity and efficiency of high-energy-density batteries.
Charge-Discharge Cycles: The number of times a battery goes through charge and discharge cycles, also known as the depth of cycling, affects its performance. Repeated cycling can cause the growth of solid electrolyte interface (SEI) layers, which can increase the battery's internal resistance and reduce its capacity over time. It is important to carefully manage the depth of cycling to minimise the detrimental effects of SEI layer formation and maximise the battery's lifespan.
Electrode Design: The design and composition of the electrodes in high-energy-density batteries also significantly impact their performance. The choice of materials for the positive and negative electrodes can determine the battery's energy density, power density, and cycling stability. Researchers often explore electrode materials, such as lithium-based compounds or transition metal oxides, to improve the battery's performance. Additionally, the structural design of the electrodes, including their morphology and particle size, can influence the battery's charge-discharge efficiency and overall performance.
Overall, optimising temperature conditions, carefully managing charge-discharge cycles, and implementing advanced electrode designs are all crucial for enhancing the performance of high-energy-density batteries. Understanding these factors and continuously improving battery technologies can pave the way towards more efficient and sustainable energy storage solutions.
What are the potential environmental benefits of using high-energy-density batteries with improved efficiency and energy-to-weight ratio?
High energy density batteries with improved efficiency and energy-to-weight ratio offer several potential environmental benefits.
Reduced Emissions: These advanced batteries have the potential to reduce greenhouse gas emissions by minimising the reliance on fossil fuels. By enabling the effective storage and utilisation of renewable energy sources such as solar and wind power, these batteries contribute to a cleaner and more sustainable energy system.
Lower Carbon Footprint: High energy density batteries can enhance the efficiency of electric vehicles, which leads to lower carbon emissions compared to traditional internal combustion engine vehicles. By transitioning to electric vehicles powered by these batteries, we can significantly reduce the carbon footprint associated with transportation.
Decreased Air Pollution: The use of high energy density batteries can also lead to a reduction in air pollution. By replacing vehicles and equipment that run on gasoline or diesel with electric alternatives, we can mitigate the emissions of harmful pollutants such as nitrogen oxides and particulate matter, improving air quality and public health.
Resource Conservation: These batteries offer an improved energy-to-weight ratio, meaning they can store more energy with less bulk and weight. This not only increases the overall efficiency of energy storage systems but also helps conserve natural resources. These batteries preserve valuable resources by requiring fewer raw materials for their production.
Waste Reduction: High energy density batteries have a longer lifespan and improved durability. This reduces the number of batteries that need to be discarded and disposed of, thereby minimising the environmental impact of battery waste. Additionally, advancements in battery technology have enabled better recycling methods, allowing for the recovery of valuable materials and further reducing waste.
Conclusion
Using high energy density batteries with improved efficiency and energy-to-weight ratio presents significant environmental benefits, including reduced emissions, lower carbon footprint, decreased air pollution, resource conservation, and waste reduction. By adopting these batteries and integrating them into various applications, we can contribute to a greener and more sustainable future.
Comments